Superconducting nanowire single-photon detector
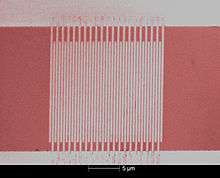
The superconducting nanowire single-photon detector (SNSPD) is a type of near-infrared and optical single-photon detector based on a current-biased superconducting nanowire.[1] It was first developed by scientists at Moscow State Pedagogical University and at the University of Rochester in 2001.[2][3]
As of 2013, a superconducting nanowire single-photon detector is the fastest single-photon detector (SPD) for photon counting.[4]
Principle of operation
The SNSPD consists of a thin (≈ 5 nm) and narrow (≈ 100 nm) superconducting nanowire. The length is typically hundreds of micrometers, and the nanowire is patterned in a compact meander geometry to create a square or circular pixel with high detection efficiency. The nanowire is cooled well below its superconducting critical temperature and biased with a DC current that is close to but less than the superconducting critical current of the nanowire. A photon incident on the nanowire breaks Cooper pairs and reduces the local critical current below that of the bias current. This results in the formation of a localized non-superconducting region, or hotspot, with finite electrical resistance. This resistance is typically larger than the 50 ohm input impedance of the readout amplifier, and hence most of the bias current is shunted to the amplifier. This produces a measurable voltage pulse that is approximately equal to the bias current multiplied by 50 ohms. With most of the bias current flowing through the amplifier, the non-superconducting region cools and returns to the superconducting state. The time for the current to return to the nanowire is typically set by the inductive time constant of the nanowire, equal to the kinetic inductance of the nanowire divided by the impedance of the readout circuit.[5] Proper self-resetting of the device requires that this inductive time constant be slower than the intrinsic cooling time of the nanowire hotspot.[6]
While the SNSPD does not offer the intrinsic energy or photon-number resolution of the superconducting transition edge sensor, the SNSPD is significantly faster than conventional transition edge sensors and operates at higher temperatures. Most SNSPDs are made of niobium nitride (NbN), which offers a relatively high superconducting critical temperature (≈ 10 K) and a very fast cooling time (<100 picoseconds).[7] NbN devices have demonstrated device detection efficiencies as high as 67% at 1064 nm wavelength with count rates in the hundreds of MHz.[8] NbN devices have also demonstrated jitter – the uncertainty in the photon arrival time – of less than 50 picoseconds,[9] as well as very low rates of dark counts, i.e. the occurrence of voltage pulses in the absence of a detected photon.[10] In addition, the deadtime (time interval following a detection event during which the detector is not sensitive) is on the order of a few nanoseconds, this short deadtime translates into very high saturation count rates and enables antibunching measurements with a single detector.[11]
For the detection of longer wavelength photons, however, the detection efficiency of standard SNSPDs decreases significantly.[12] Recent efforts to improve the detection efficiency at near-infrared and mid-infrared wavelengths include studies of narrower (20 nm and 30 nm wide) NbN nanowires[13] as well as studies of materials with lower superconducting critical temperatures than NbN (tungsten silicide,[14] niobium silicide,[15] and tantalum nitride).
Applications
Many of the initial application demonstrations of SNSPDs have been in the area of quantum information, such as quantum key distribution[16] and quantum computing.[17] Other applications include imaging of infrared photoemission for defect analysis in CMOS circuitry,[18] LIDAR,[19] on-chip quantum optics,[20] single plasmon detection,[21] quantum plasmonics,[22] single electron detection,[23] single α and β particles detection,[24] oxygen singlet luminescence detection[25] and ultra-long distance classical communication.[26] A number of companies are commercializing complete single photon detection systems based on superconducting nanowires, including Scontel, Photon Spot, Single Quantum, and Quantum Opus.
References
- ↑ C. M. Natarajan, M. G. Tanner, and R. H. Hadfield, "Superconducting nanowire single-photon detectors: physics and applications," Superconductor Science and Technology 25, 063001 (2012), doi:10.1088/0953-2048/25/6/063001, arXiv:1204.5560
- ↑ A. D. Semenov, G. N. Gol’tsman and A. A. Korneev, "Quantum detection by current carrying superconducting film," Physica C 351, 349 (2001), doi:10.1016/S0921-4534(00)01637-3
- ↑ G. N. Gol'tsman et al., "Picosecond superconducting single-photon optical detector," Applied Physics Letters 79, 705 (2001), doi:10.1063/1.1388868
- ↑ Francesco Marsili. "High Efficiency in the Fastest Single-Photon Detector System". 2013.
- ↑ A. J. Kerman et al., "Kinetic-inductance-limited reset time of superconducting nanowire photon counters," Applied Physics Letters 88, 111116 (2006), doi:10.1063/1.2183810, arXiv:0510238
- ↑ A. J. Annunziata et al., "Reset dynamics and latching in niobium superconducting nanowire single photon detectors," Journal of Applied Physics 108, 084507 (2010), doi:10.1063/1.3498809, arXiv:1008.0895
- ↑ Yu. P. Gousev et al., "Electron-phonon interaction in disordered NbN films," Physica B 194-196, 1355 (1994), doi:10.1016/0921-4526(94)91007-3
- ↑ K. M. Rosfjord et al., "Nanowire single-photon detector with an integrated optical cavity and anti-reflection coating," Optics Express 14, 527 (2006), doi:10.1364/OPEX.14.000527
- ↑ J. Zhang et al., "Response time characterization of NbN superconducting single-photon detectors," IEEE Transactions on Applied Superconductivity 13, 180 (2003), doi:10.1109/TASC.2003.813675
- ↑ J. Kitaygorsky et al., "Origin of dark counts in nanostructured NbN single-photon detectors," IEEE Transactions on Applied Superconductivity 15, 545 (2005), doi:10.1109/TASC.2005.849914
- ↑ G. A. Steudleet al., "Measuring the quantum nature of light with a single source and a single detector," Physical Review A 86, 053814 (2012), doi:10.1103/PhysRevA.86.053814
- ↑ A. Korneev et al., "Quantum efficiency and noise equivalent power of nanostructured NbN single-photon detectors in the wavelength range from visible to infrared," IEEE Transactions on Applied Superconductivity 15, 571 (2005), doi:10.1109/TASC.2005.849923
- ↑ F. Marsili et al., "Single-photon detectors based on ultranarrow superconducting nanowires," Nano Letters 11, 2048 (2011), doi:10.1021/nl2005143, arXiv:1012.4149
- ↑ B. Baek, A. E. Lita, V. Verma and S. W. Nam, "Superconducting a-WxSi1−x nanowire single-photon detector with saturated internal quantum efficiency from visible to 1850 nm," Applied Physics Letters 98, 251105 (2011), doi:10.1063/1.3600793
- ↑ S. N. Dorenbos et al., "Low gap superconducting single photon detectors for infrared sensitivity," Applied Physics Letters 98, 251102 (2011), doi:10.1063/1.3599712
- ↑ H. Takesue et al., "Quantum key distribution over a 40-dB channel loss using superconducting single-photon detectors," Nature Photonics 1, 343 (2007), doi:10.1038/nphoton.2007.75, arXiv:0706.0397
- ↑ R. H. Hadfield, "Single-photon detectors for optical quantum information applications," Nature Photonics 3, 696 (2009), doi:10.1038/nphoton.2009.230
- ↑ M. K. McManus et al., "PICA: Backside failure analysis of CMOS circuits using picosecond imaging analysis," Microelectronics Reliability 40, 1353 (2000), doi:10.1016/S0026-2714(00)00137-2
- ↑ A. Mc Carthy et al., "Kilometer-range, high resolution depth imaging via 1560 nm wavelength single-photon detection," Optics Express21, 8904 (2013), doi:10.1364/OE.21.008904
- ↑ G. Reithmaier et al., "On-chip generation, routing and detection of quantum light," (2014), arXiv:1408.2275v2
- ↑ R. W. Heeres et al., "On-chip single plasmon detection," Nanoletters10, 661(2012), doi:10.1021/nl903761t
- ↑ R. W. Heeres et al., "Quantum interference of surface plasmons," Nature Nanotechnology 8, 719 (2013), doi:10.1038/nnano.2013.150
- ↑ M. Rosticher et al., "A high efficiency superconducting nanowire single electron detector," Applied Physics Letters 97, 183106 (2010), doi:10.1063/1.3506692
- ↑ H. Azzouz et al., "Efficient single particle detection with a superconducting nanowire," AIP Advances 2, 032124 (2012), doi:10.1063/1.4740074
- ↑ N. R. Gemmell et al., "Singlet oxygen luminescence detection with a fiber-coupled superconducting nanowire single-photon detector," Optics Express 21, 5005(2013), doi:10.1364/OE.21.005005
- ↑ D. M. Boroson, R. S. Bondurant and J. J. Scozzafava, "Overview of high rate deep space laser communications options," Proc. SPIE 5338, 37 (2004), doi:10.1117/12.543010