Sound localization in owls
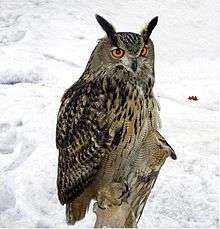
Most owls are nocturnal or crepuscular birds of prey. Because they hunt at night, they must rely on non-visual senses. Experiments by Roger Payne[1] have shown that owls are sensitive to the sounds made by their prey, not the heat or the smell. In fact, the sound cues are both necessary and sufficient for localization of mice from a distant location where they are perched. For this to work, the owls must be able to accurately localize both the azimuth and the elevation of the sound source.
ITD and ILD
Owls must be able to determine the necessary angle of descent, i.e. the elevation, in addition to azimuth (horizontal angle to the sound). This bi-coordinate sound localization is accomplished through two binaural cues: the interaural time difference (ITD) and the interaural level difference (ILD), also known as the interaural intensity difference (IID). The ability in owls is unusual; in ground-bound mammals such as mice, ITD and ILD are not utilized in the same manner. In these mammals, ITDs tend to be utilized for localization of lower frequency sounds, while ILDs tend to be used for higher frequency sounds.
ITD occurs whenever the distance from the source of sound to the two ears is different, resulting in differences in the arrival times of the sound at the two ears. When the sound source is directly in front of the owl, there is no ITD, i.e. the ITD is zero. In sound localization, ITDs are used as cues for location in the azimuth. ITD changes systematically with azimuth. Sounds to the right arrive first at the right ear; sounds to the left arrive first at the left ear.
In mammals there is a level difference in sounds at the two ears caused by the sound-shadowing effect of the head. But in many species of owls, level differences arise primarily for sounds that are shifted above or below the elevation of the horizontal plane. This is due to the asymmetry in placement of the ear openings in the owl's head, such that sounds from below the owl reach the left ear first and sounds from above reach the right ear first.[2] IID is a measure of the difference in the level of the sound as it reaches each ear. In many owls, IIDs for high-frequency sounds (higher than 4 or 5 kHz) are the principal cues for locating sound elevation.
Parallel processing pathways in the brain
The axons of the auditory nerve originate from the hair cells of the cochlea in the inner ear. Different sound frequencies are encoded by different fibers of the auditory nerve, arranged along the length of the auditory nerve, but codes for the timing and level of the sound are not segregated within the auditory nerve. Instead, the ITD is encoded by phase locking, i.e. firing at or near a particular phase angle of the sinusoidal stimulus sound wave, and the IID is encoded by spike rate. Both parameters are carried by each fiber of the auditory nerve.[3]
The fibers of the auditory nerve innervate both cochlear nuclei in the brainstem, the cochlear nucleus magnocellularis (mammalian anteroventral cochlear nucleus) and the cochlear nucleus angularis (see figure; mammalian posteroventral and dorsal cochlear nuclei). The neurons of the nucleus magnocellularis phase-lock, but are fairly insensitive to variations in sound pressure, while the neurons of the nucleus angularis phase-lock poorly, if at all, but are sensitive to variations in sound pressure. These two nuclei are the starting points of two separate but parallel pathways to the inferior colliculus: the pathway from nucleus magnocellularis processes ITDs, and the pathway from nucleus angularis processes IID.

In the time pathway, the nucleus laminaris (mammalian medial superior olive) is the first site of binaural convergence. It is here that ITD is detected and encoded using neuronal delay lines and coincidence detection, as in the Jeffress model; when phase-locked impulses coming from the left and right ears coincide at a laminaris neuron, the cell fires most strongly. Thus, the nucleus laminaris acts as a delay-line coincidence detector, converting distance traveled to time delay and generating a map of interaural time difference. Neurons from the nucleus laminaris project to the core of the central nucleus of the inferior colliculus and to the anterior lateral lemniscal nucleus.
In the sound level pathway, the posterior lateral lemniscal nucleus (mammalian lateral superior olive) is the site of binaural convergence and where IID is processed. Stimulation of the contralateral ear inhibits and that of the ipsilateral ear excites the neurons of the nuclei in each brain hemisphere independently. The degree of excitation and inhibition depends on sound pressure, and the difference between the strength of the inhibitory input and that of the excitatory input determines the rate at which neurons of the lemniscal nucleus fire. Thus the response of these neurons is a function of the difference in sound pressure between the two ears.
The time and sound-pressure pathways converge at the lateral shell of the central nucleus of the inferior colliculus. The lateral shell projects to the external nucleus, where each space-specific neuron responds to acoustic stimuli only if the sound originates from a restricted area in space, i.e. the receptive field of that neuron. These neurons respond exclusively to binaural signals containing the same ITD and IID that would be created by a sound source located in the neuron’s receptive field. Thus their receptive fields arise from the neurons’ tuning to particular combinations of ITD and IID, simultaneously in a narrow range. These space-specific neurons can thus form a map of auditory space in which the positions of receptive fields in space are isomorphically projected onto the anatomical sites of the neurons.[4]
Significance of asymmetrical ears for localization of elevation
The ears of many species of owls are asymmetrical. For example, in barn owls (Tyto alba), the placement of the two ear flaps (operculi) lying directly in front of the ear canal opening is different for each ear. This asymmetry is such that the center of the left ear flap is slightly above a horizontal line passing through the eyes and directed downward, while the center of the right ear flap is slightly below the line and directed upward. In two other species of owls with asymmetrical ears, the saw-whet owl and the long-eared owl, the asymmetry is achieved by different means: in saw whets, the skull is asymmetrical; in the long-eared owl, the skin structures lying near the ear form asymmetrical entrances to the ear canals, which is achieved by a horizontal membrane. Thus, ear asymmetry seems to have evolved on at least three different occasions among owls. Because owls depend on their sense of hearing for hunting, this convergent evolution in owl ears suggests that asymmetry is important for sound localization in the owl.
Ear asymmetry allows for sound originating from below the eye level to sound louder in the left ear, while sound originating from above the eye level to sound louder in the right ear. Asymmetrical ear placement also causes IID for high frequencies (between 4 kHz and 8 kHz) to vary systematically with elevation, converting IID into a map of elevation. Thus, it is essential for an owl to have the ability to hear high frequencies. Many birds have the neurophysiological machinery to process both ITD and IID, but because they have small heads and low frequency sensitivity, they use both parameters only for localization in the azimuth. Through evolution, the ability to hear frequencies higher than 3 kHz, the highest frequency of owl flight noise, enabled owls to exploit elevational IIDs, produced by small ear asymmetries that arose by chance, and began the evolution of more elaborate forms of ear asymmetry.[5]
Another demonstration of the importance of ear asymmetry in owls is that, in experiments, owls with symmetrical ears, such as the screech owl (Otus asio) and the great horned owl (Bubo virginianus), could not be trained to locate prey in total darkness, whereas owls with asymmetrical ears could be trained.[6]
References
- ↑ Payne, Roger S., 1962. How the Barn Owl Locates Prey by Hearing. The Living Bird, First Annual of the Cornell Laboratory of Ornithology, 151-159
- ↑ Knudsen EI. 1981. The hearing of the barn owl. Sci Am 245(6):113-25
- ↑ Zupanc, Gunther K.H. Behavioral Neurobiology: An integrative approach. Oxford University Press, New York: 2004, 142-149
- ↑ Knudsen, Eric I and Masakazu Konishi. A Neural Map of Auditory Space in the Owl. Science. Vol. 200, 19 May 1978: 795-797
- ↑ Konishi, Masakazu and Susan F. Volman, 1994. Adaptations for bi-coordinate sound localization in owls. Neural Basis of Behavioral Adaptations, 1-9
- ↑ Payne, Roger S.. Acoustic Location of Prey by Barn Owls (Tyto alba). J Exp Biol. 1971; 54, 535-573